Gibberellins
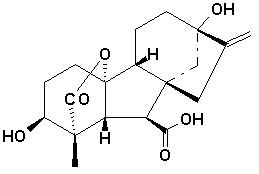
Nature of Gibberellins
Unlike the classification of auxins which are classified on the basis of function, gibberellins are classified on the basis of structure as well as function. All gibberellins are derived from the ent-gibberellane skeleton. The structure of this skeleton derivative along with the structure of a few of the active gibberellins are shown above. The gibberellins are named GA1....GAn in order of discovery. Gibberellic acid, which was the first gibberellin to be structurally characterised , is GA3.
There are currently 136 GAs identified from plants, fungi and bacteria.
GA's are widespread and so far ubiquitous in both flowering (angiosperms) and non-flowering (gymnosperms) plants as well as ferns.
History of Gibberellins
Gibberellins (GAs) are a group of diterpenoid acids that function as plant growth regulators inflencing a range of developmental processes in higher plants including stem elongation, germination, dormancy, flowering, sex expression, enzyme induction and leaf and fruit senescence. The origin of research into gibberellins can be traced to Japanese plant pathologists who were investigating the causes of the "bakanae" (foolish seedling) disease which seriously lowered the yield of rice crops in Japan, Taiwan and throughout the Asian continent.
Symptoms of the disease are pale yellow, elongated seedlings with slender leaves and stunted roots. Severely diseased plants die whereas plants with slight symptoms survive but produce poorly developed grain, or none at all.
Bakanae is now easily prevented by treatment of seeds with fungicides prior to sowing. The first paper on the cause of bakanae was published in 1898 by Shotaro Hori who demonstrated that the symptoms were induced by infection with a fungus belonging to the genus Fusarium, probably Fusarium heterosporium Nees.

In 1912, Sawada published a paper in the Formosan Agricultural Review entitled "The Diseases of Crops in Taiwan" in which he suggested that the elongation in rice seedlings infected with bakanae fungus might be due to a stimulus derived from fungal hyphae.
Subsequently, Eiichi Kurosawa (1926) found that culture filtrates from dried rice seedlings caused marked elongation in rice and other sub-tropical grasses. He concluded that bakanae fungus secretes a chemical that stimulates shoot elongation, inhibits chlorophyll formation and suppresses root growth.
Although there has been controversy among plant pathologists over the nomenclature of bakanae fungus, in the 1930s, the imperfect stage of the fungus was named Fusarium moniliforme (Sheldon) and the perfect stage, Gibberella fujikuroi (Saw.) Wr. by H.W. Wollenweber. The terms "Fujikuroi" and "Saw." in Gibberella fujikuroi (Saw.) Wr. were derived from the names of two distinguished Japanese plant pathologists, Yosaburo Fujikuro and Kenkichi Sawada.
Teijiro Yabuta initiated work on the isolation of the active component using the fungal strains provided by Kurosawa. In 1934, Yabuta isolated a crystalline compound from the fungal culture filtrate that inhibited growth of rice seedlings at all concentrations tested. The structure of the inhibitor was shown to be 5-n-butylpicolinic acid or fusaric acid. The formation of fusaric acid in culture filtrates was suppressed by changing the composition of the culture medium. As a result, a non-crystalline solid was obtained from the culture filtrate that stimulated the growth of rice seedlings. This compound was named gibberellin by Yabuta in 1935; the first use of the term "gibberellin" in the scientific literature.
In 1938, Yabuta and his associate Yusuke Sumiki finally succeeded in crystallizing a pale yellow solid to yield gibberellin A and gibberellin B (The names were subsequently interchanged in 1941 and the original gibberellin A was found to be inactive.) Determination of the structure of the active gibberellin was hampered by a shortage of pure crystalline sample. By current standards the productivity of their fungal strain was extremely poor and they did not know that their sample of gibberellin A was not pure, but a mixture of structurally-related gibberellins.
In the United States, the first research on gibberellins began after the Second World War by a research unit at Camp Dietrick, Maryland. In 1950, John E. Mitchell reported optimal fermentation procedures for the fungus, as well as the effects of fungal extracts on the growth of bean (Vicia faba)seedlings(Mitchell & Angel 1951). Work also began at the Northern USDA Regional Research Laboratories in Peoria, Illinois in the USA using the strain provided by Mitchell. Large scale fermentations were carried out with the purpose of producing pure gibberellin A for agricultural uses but initial fermentations were inactive.
In 1951, Sumiki visited the United States and met Frank H. Stodola. After returning to Japan he sent new cultures to the USA but these also proved inactive. The problem was traced to the lack of magnesium in the culture medium and good yields of gibberellin were obtained when the culture medium was supplemented with magnesium sulphate. The physical properties of gibberellin isolated from these fermentations were found to be surprisingly different from those reported by the Japanese and the new compound was named gibberellin-x. (Stodola et al., 1955).
At about the same time in the UK, a team of researchers ( Philip Curtis, Brian Cross, John Grove, Jake MacMillan and Paddy Mulholland) at Akers Research Laboratories (ICI) isolated a new gibberellin which was given the name "gibberellic acid".This compound had physical properties different from the Japanese gibberellin A(Curtis & Cross, 1954). Samples were exchanged between Stodola and Grove and "gibberellic acid" and gibberellin-X were found to have identical chemical and physical properties and the name gibberellic acid was accepted by both groups. A structure for gibberellic acid was proposed in 1956 but later revised see Grove 1961.
In 1955, members of Sumuki group, (Takahashi et al.) succeeded in separating the methyl ester of gibberellin A into three components, from which corresponding free acids were obtained and named gibberellins A1, A2, and A3. Gibberellin A3 was found to be identical to gibberellic acid. In 1957, Takahashi et al. isolated a new gibberellin named gibberellin A4 as a minor component from the culture filtrate.
In the mid 1950s, evidence that gibberellins were naturally occurring substances in higher plants began to appear in the literature. Using techniques that had been used to isolate gibberellins from the fungus, Margaret Radley at ICI in the UK demonstrated the presence of gibberellin-like substances in higher plants. In the USA, the first reports of a gibberellin-like substance in maize came from Bernard Phinney et al using dwarf maize mutants to assay for activity in plant extracts. This was followed by the isolation of crystalline gibberellin A1, A5, A6 and A8 from runner bean (Phaseolus multiflorus) (MacMillan et al. 1958, 1959, 1960,1962). The original samples from these isolations are now on display at Long Ashton.
In the 1960s the number of gibberellins reported in the literature isolated from fungal and plant origins rapidly increased. In 1968, J. MacMillan & N. Takahashi reached an agreement that all gibberellins should be assigned numbers as gibberellin A1-x, irrespective of their origin. Over the past 20 years using modern analytical techniques many more gibberellins have been identified. At the present time the current number of gibberellins identified is 126.
For a more detailed description of the history of gibberellins please see Phinney, B.O.(1983) and Tamura, S. (1990)
Gibberellin Biosynthesis and Metabolism
Gibberellins are diterpenes synthesized from acetyl CoA via the mevalonic acid pathway. They all have either 19 or 20 carbon units grouped into either four or five ring systems. The fifth ring is a lactone ring as shown in the structures above attached to ring A. Gibberellins are believed to be synthesized in young tissues of the shoot and also the developing seed. It is uncertain whether young root tissues also produce gibberellins. There is also some evidence that leaves may be the source of some biosynthesis (Sponsel, 1995; Salisbury and Ross). The pathway by which gibberellins are formed is outlined below.
- 3 acetyl CoA molecules are oxidized by 2 NADPH molecules to produce 3 CoA molecules as a side product and mevalonic acid.
- Mevalonic acid is then Phosphorylated by ATP and decarboxylated to form isopentyl pyrophosphate.
- 4 of these molecules form geranylgeranyl pyrophosphate which serves as the donor for all GA carbon atoms.
- This compound is then converted to copalylpyrophosphate which has 2 ring systems.
- Copalylpyrophosphate is then converted to kaurene which has 4 ring systems.
- Subsequent oxidations reveal kaurenol (alcohol form), kaurenal (aldehyde form), and kaurenoic acid respectively.
- Kaurenoic acid is converted to the aldehyde form of GA12 by decarboxylation. GA12 is the 1st true gibberellane ring system with 20 carbons.
- From the aldehyde form of GA12 arise both 20 and 19 carbon gibberellins but there are many mechanisms by which these other compounds arise.
Certain commercial chemicals which are used to stunt growth do so in part because they block the synthesis of gibberellins. Some of these chemicals are Phosphon D, Amo-1618, Cycocel (CCC), ancymidol, and paclobutrazol. During active growth, the plant will metabolize most gibberellins by hydroxylation to inactive conjugates quickly with the exception of GA3. GA3 is degraded much slower which helps to explain why the symptoms initially associated with the hormone in the disease bakanae are present. Inactive conjugates might be stored or translocated via the phloem and xylem before their release (activation) at the proper time and in the proper tissue (Arteca, 1996; Sponsel, 1995).
Functions of Gibberellins
Active gibberellins show many physiological effects, each depending on the type of gibberellin present as well as the species of plant. Some of the physiological processes stimulated by gibberellins are outlined below (Davies, 1995; Mauseth, 1991; Raven, 1992; Salisbury and Ross, 1992).
- Stimulates stem elongation by stimulating cell division and elongation.
- Stimulates bolting/flowering in response to long days.
- Breaks seed dormancy in some plants which require stratification or light to induce germination.
- Stimulates enzyme production (a-amylase) in germinating cereal grains for mobilization of seed reserves.
- Induces maleness in dioecious flowers (sex expression).
- Can cause parthenocarpic (seedless) fruit development.
- Can delay senescence in leaves and citrus fruits.